- Published 23 May 2024
- Last Modified 23 May 2024
- 6 min
Silicon Carbide Semiconductor Guide
This guide will explain the material’s high applicability to semiconductor devices.
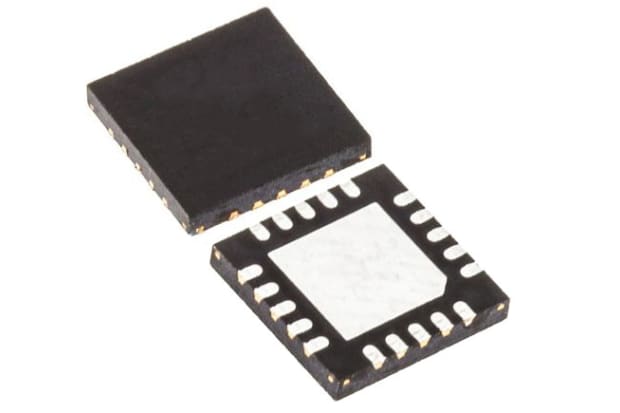
Silicon faces stiff competition from silicon carbide in the race for the title of the ideal semiconductor material. Silicon carbide boasts superior thermal conductivity, high temperature and high voltage capabilities, breakdown voltage, and energy gap compared to silicon. This guide aims to elucidate the material's extensive applicability to semiconductor devices in New Zealand.
Exploring Semiconductors: Silicon vs. Silicon Carbide
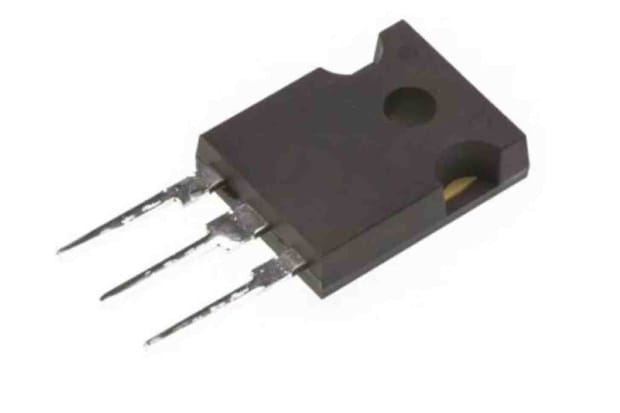
Semiconductors are the backbone of electronic devices that power our interconnected world. While silicon (Si) has traditionally dominated this arena, a formidable contender has emerged in the form of silicon carbide (SiC).
Semiconductor materials possess unique properties, including a conductivity level between that of insulators and conductors, making them versatile for various electronic applications. They also exhibit low resistivity at elevated temperatures, making them suitable for use in computer chips.
These materials' properties can be finely tuned by introducing impurities into their molecular structure, a process known as doping. This modification alters the paths for electron flow within silicon and silicon carbide, enabling customization for specific electronic applications.
SiC devices outshine Si ones in many sought-after semiconductor properties, positioning them as key players in the pursuit of greater efficiencies and performance enhancements.
Unveiling Silicon Carbide Properties
The properties of silicon carbide (SiC) distinguish it from pure silicon due to its unique atomic structure. While silicon forms bonds with four adjacent silicon atoms in a basic grid, silicon bonded with carbon creates tightly packed tetrahedrons consisting of four carbon atoms with a silicon atom in the middle. This crystalline structure maximizes power density, efficiency, and reliability.
Thermal Conductivity
Thermal conductivity measures how effectively heat can traverse through a material, a critical property for semiconductors. It indicates how efficiently materials dissipate heat, crucial for managing voltage and current capacities as power increases with higher currents.
Silicon's thermal conductivity of 130 W/(m⋅K) pales in comparison to silicon carbide's impressive 490 W/(m⋅K). This superior thermal conductivity enables silicon carbide semiconductors to efficiently dissipate heat from higher operating voltages.
Thermal Expansion
Thermal expansion occurs when a material changes its shape or size due to temperature fluctuations without changing phase. Silicon carbide boasts a relatively low coefficient of thermal expansion, allowing it to maintain its shape, strength, and performance at higher temperatures and voltages better than silicon.
Electric Field Strength
Two crucial semiconductor properties, closely related to each other, are the material's band gap and maximum electric field strength.
In semiconductor materials, electrons transition between different energy bands. The band gap represents the energy required for electrons to move from the valence band to the conduction band, facilitating electrical conductivity. When semiconductors receive electrical energy and enter this conductive state, they showcase their unique insulator/conductor hybrid nature.
Silicon carbide semiconductors possess an energy gap three times higher than silicon-based ones, enabling them to withstand higher electric field strengths and operate at elevated voltages and temperatures.
Advantages of Silicon Carbide Semiconductors
Silicon carbide semiconductors offer a host of advantages over their silicon-based counterparts, as highlighted below:
Larger Energy Gap
Silicon carbide's larger energy gap proves highly advantageous in higher-power applications. A larger energy gap allows semiconductor devices to be smaller while maintaining higher performance levels.
In diodes, which are among the most common semiconductor components, the breakdown voltage denotes the voltage at which reverse-applied current can flow through the diode. Silicon carbide's high breakdown voltage renders them a superior choice for MOSFETs (Metal-Oxide-Semiconductor Field-Effect Transistors).
Another crucial property for semiconductors in MOSFETs is the reverse recovery time. This refers to the time taken by MOSFETs to return to their normal state after entering a reverse-biased state. During this period, the current can flow in reverse, leading to energy losses in the system. SiC devices exhibit extremely fast reverse recovery times and minimal energy losses compared to Si devices.
Silicon carbide offers more versatility in doping (the addition of impurities) compared to silicon. It can be tailored to conduct only under specific conditions, such as when exposed to certain intensities of light (infrared, visible, or ultraviolet). This versatility enhances the applications of silicon carbide semiconductors, expanding their utility in various fields.
Diverse Applications of Silicon Carbide Semiconductor Devices
Silicon carbide semiconductor devices find application across a wide spectrum:
MOSFETs (Metal Oxide Semiconductor Field-Effect Transistors)
MOSFETs are discrete semiconductor devices controlled by a capacitor. They regulate current flow from the source to drain terminals, offering potential for signal amplification and switching. Silicon carbide's high breakdown voltage and low reverse recovery time make it an optimal choice for MOSFET design.
Schottky and Rectifier Diodes
Schottky diodes exhibit rapid switching within nanoseconds and feature a low forward voltage drop, enhancing system efficiency. They are employed in various applications to prevent accidental discharge from devices like mains-connected batteries and solar panel cells. Rectifier diodes facilitate current flow in a single direction, converting alternating current into direct current. In both diode applications, silicon carbide offers superior performance and higher voltage protection against faults, spikes, and surges.
Discover Schottky Diodes & Rectifiers
Semiconductor Kits and Modules
RS also offers semiconductor kit and module solutions, simplifying the integration of SiC devices into operational setups.
These powerful yet compact SiC devices are making significant strides in today's leading industries:
Electric Vehicles (EVs): Electric vehicles demand high performance and a small footprint for their motor drives, both of which silicon carbide semiconductors provide. SiC's fast switching speed and higher power capacities contribute to improving charging times at public charging stations.
Data Centres: Data centres, the 'clouds' we rely on for connectivity in both business and personal spheres, dissipate vast amounts of heat due to the energy they consume. The properties of SiC devices enable these systems to operate more efficiently by running cooler.
Uninterruptible Power Supplies (UPS): UPS systems[KY4] are crucial for sustaining data centres and maintaining connectivity during mains electricity outages. A UPS converts power from DC voltage to AC voltage, and SiC components in the electronics circuitry efficiently fulfill this function, allowing for higher deliverable energy and extended support time.
Browse our range of semiconductor devices to discover which silicon carbide options can enhance your business outcomes.